Bee Theory introduces a wave-based gravitational paradigm that seeks to unify the observed effects commonly attributed to dark matter and dark energy. By positing that space-time itself hosts oscillatory modes—capable of constructive and destructive interference—Bee Theory offers an unconventional lens on longstanding cosmological puzzles. Yet, every new framework must withstand intensive scrutiny. Below, we examine the principal critiques, delve into theoretical and experimental limitations, and propose potential resolutions that could shape the future trajectory of Bee Theory research.
1. Introduction
Conventional cosmology explains galactic rotation curves and large-scale acceleration via non-baryonic dark matter and dark energy, respectively. Bee Theory rejects this two-pronged approach, arguing instead that wave interference in a gravitational field can mimic these effects. However, reconciling Bee Theory with the successes of General Relativity (GR), quantum field theories, and precision cosmological data demands rigor and an open discussion of limitations. This page provides a thorough, technical exploration of the critical challenges confronting Bee Theory.
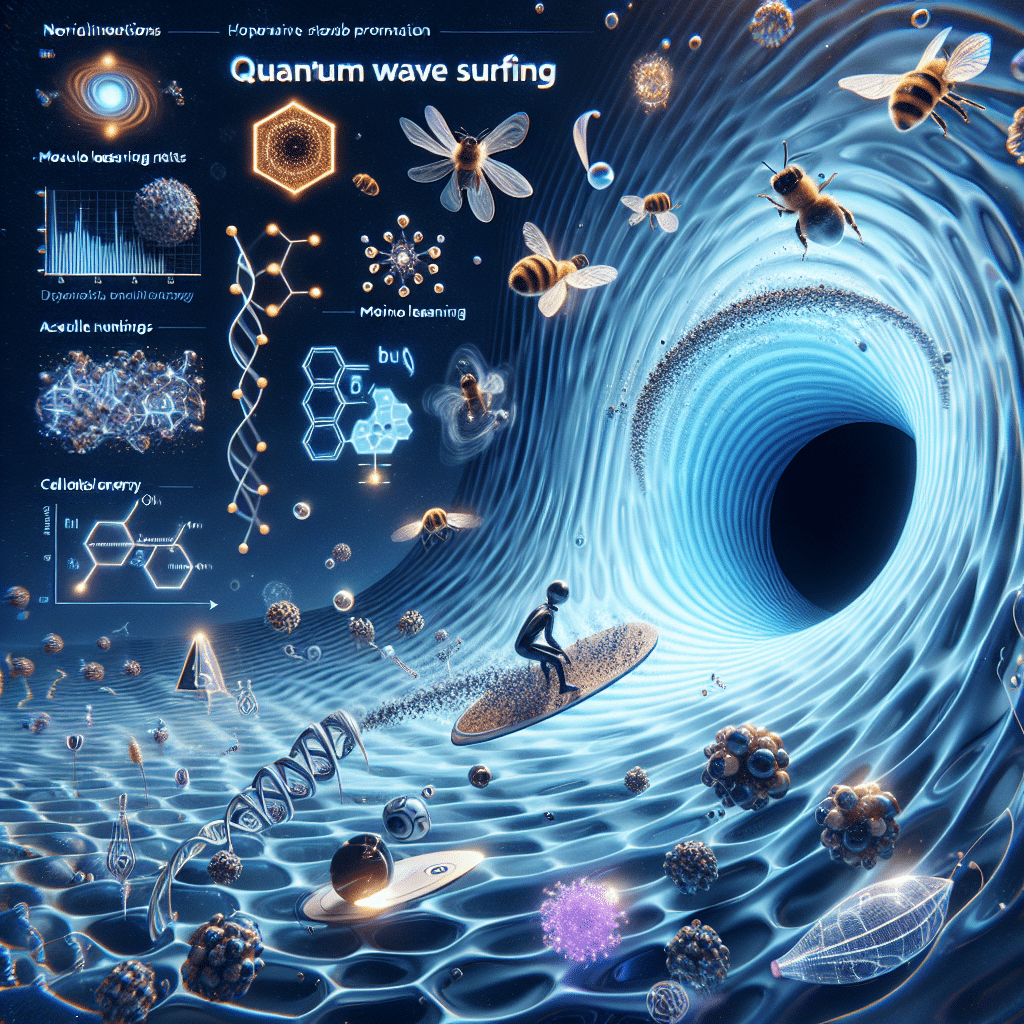
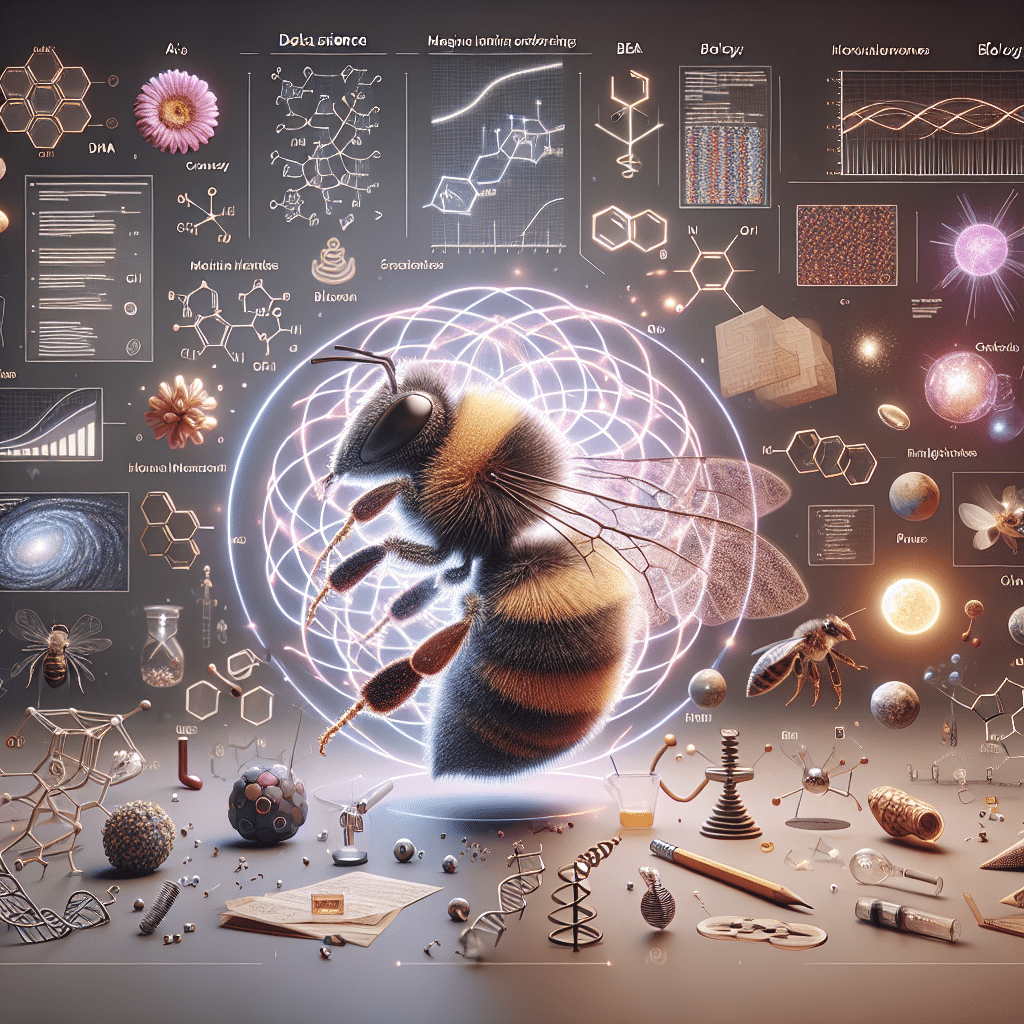
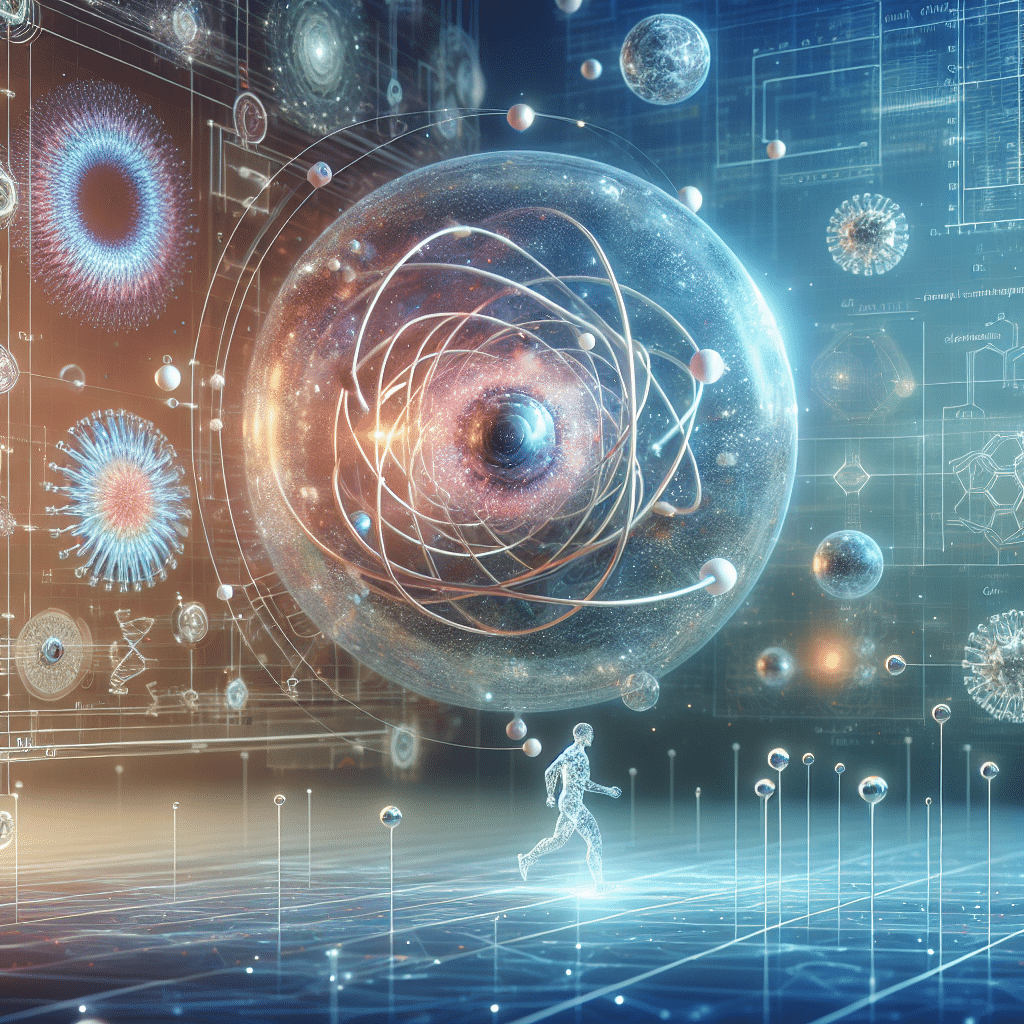
2. Major Critiques from the Scientific Community
2.1 Mathematical Rigor and Consistency
- Conflict with Einstein’s Field Equations
Many critics highlight the lack of a comprehensive mathematical framework analogous to Einstein’s equations. While Bee Theory posits emergent gravity from wave interference, it has yet to present a detailed set of field equations capable of reproducing the entire spectrum of relativistic phenomena—such as gravitational time dilation, frame dragging, and the perihelion shift of Mercury. - Comparisons with Existing Emergent Gravity Approaches
There are multiple emergent gravity proposals (e.g., Erik Verlinde’s emergent gravity or holographic approaches). Critics demand that Bee Theory clarify its distinctions and show consistent derivations for phenomena already well-explained by other emergent models. - Lack of Gauge-Invariant Formulation
In modern physics, gauge invariance is a cornerstone, ensuring physical observables are independent of arbitrary reference frames. Bee Theory’s wave description needs a robust gauge-invariant formulation or an equivalent principle that underpins its predictions, so that local observers can interpret the wave-like phenomena consistently.
2.2 Experimental Tension
- Local Tests of Gravity
Precision measurements within the Solar System (e.g., laser ranging to the Moon, tracking spacecraft trajectories around Earth and Mars) closely align with General Relativity. Bee Theory must demonstrate that wave-based modifications do not produce deviations inconsistent with these highly accurate experiments. - Binary Pulsars and Gravitational Radiation
Observations of binary pulsars show orbital decay rates matching General Relativity’s predictions for gravitational wave emission. If Bee Theory introduces additional wave modes or phase shifts, critics question whether it can reproduce these exact decay patterns without invoking ad hoc parameters. - Particle Physics Interplay
Dark matter models are closely tied to beyond-the-Standard-Model (BSM) physics—like supersymmetry or axion fields—that also address other anomalies (e.g., CP-violation, neutrino masses). Dismissing exotic particles in favor of wave interference could leave certain particle-physics puzzles unsolved, drawing skepticism from that community.
3. Identified Limitations
3.1 Theoretical Gaps
- Nonlinear Wave Equations
Bee Theory posits that gravitational interactions are the sum of oscillatory field modes. However, gravitational fields are inherently nonlinear. Crafting wave equations that remain stable and self-consistent under strong-field conditions (e.g., near black holes) is a major theoretical hurdle. - Coupling to Standard Model Fields
Gravity is universal—it couples to all forms of energy, including electromagnetic, strong, and weak interactions. Bee Theory must demonstrate how its wave-based gravitational field couples to quantum fields in a way that preserves known conservation laws, particularly energy–momentum conservation. - Quantum Gravity Consistency
At very small (Planck) scales, general relativistic concepts are expected to merge with quantum mechanics. Bee Theory, similarly, will require a quantum-consistent wave formulation—one that could, in principle, be extended or integrated with approaches like loop quantum gravity or string theory.
3.2 Observational Challenges
- Distinguishing Wave Interference from Dark Matter Halos
If wave interference patterns indeed replicate “missing mass” signals, astronomers must isolate verifiable wave-based signatures—such as quantized ring structures, resonance peaks, or phase shifts. However, complex baryonic processes (e.g., feedback from star formation) can mask these patterns. - Data Interpretation Over Long Timescales
Cosmological wave phenomena may evolve over billions of years. Long-term surveys are crucial yet difficult to coordinate. Intermittent observations risk missing subtle changes that could confirm or refute wave interference. - Dependence on High-Resolution Instrumentation
Distinguishing minor anomalies in gravitational lensing or small deviations in expansion rates demands cutting-edge telescopes (e.g., Extremely Large Telescopes, next-generation cosmic microwave background experiments) and advanced gravitational wave observatories. Funding and collaboration for these large-scale projects can pose administrative and logistical barriers.
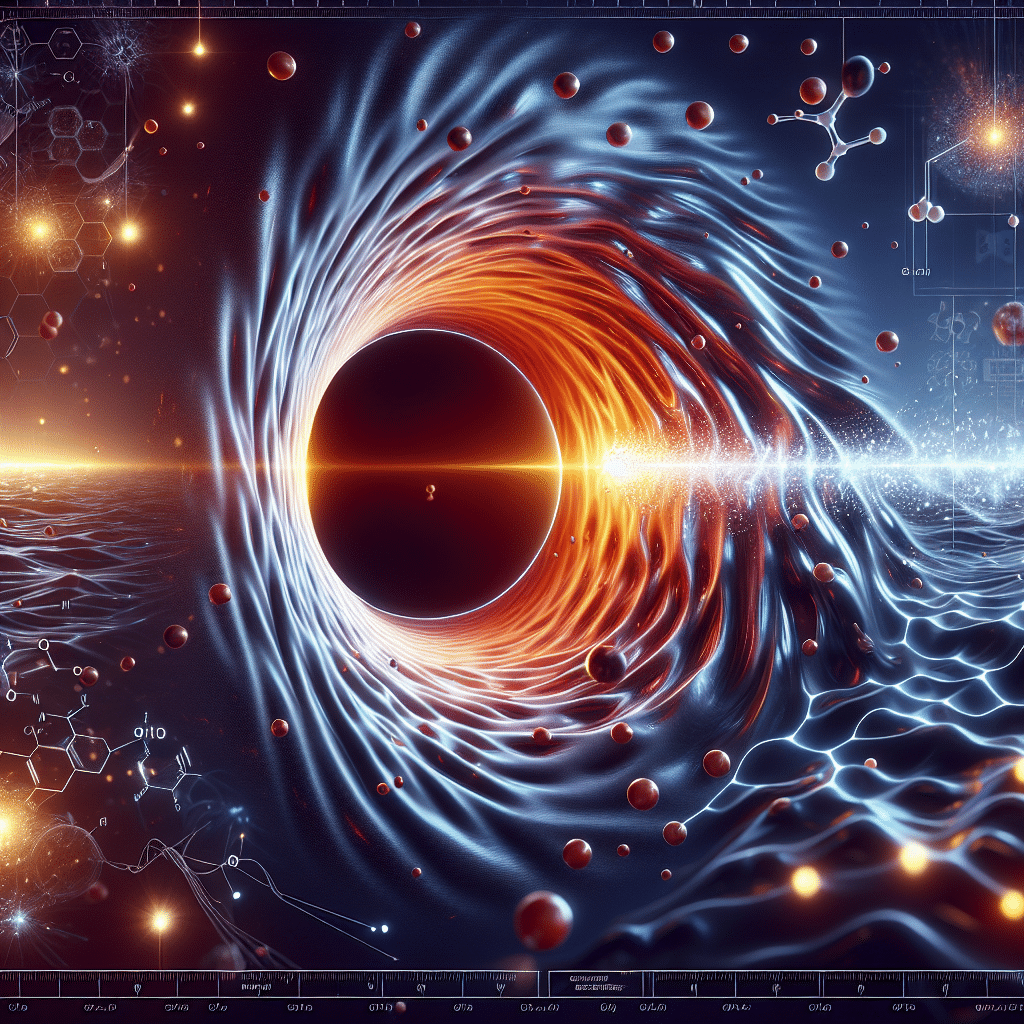
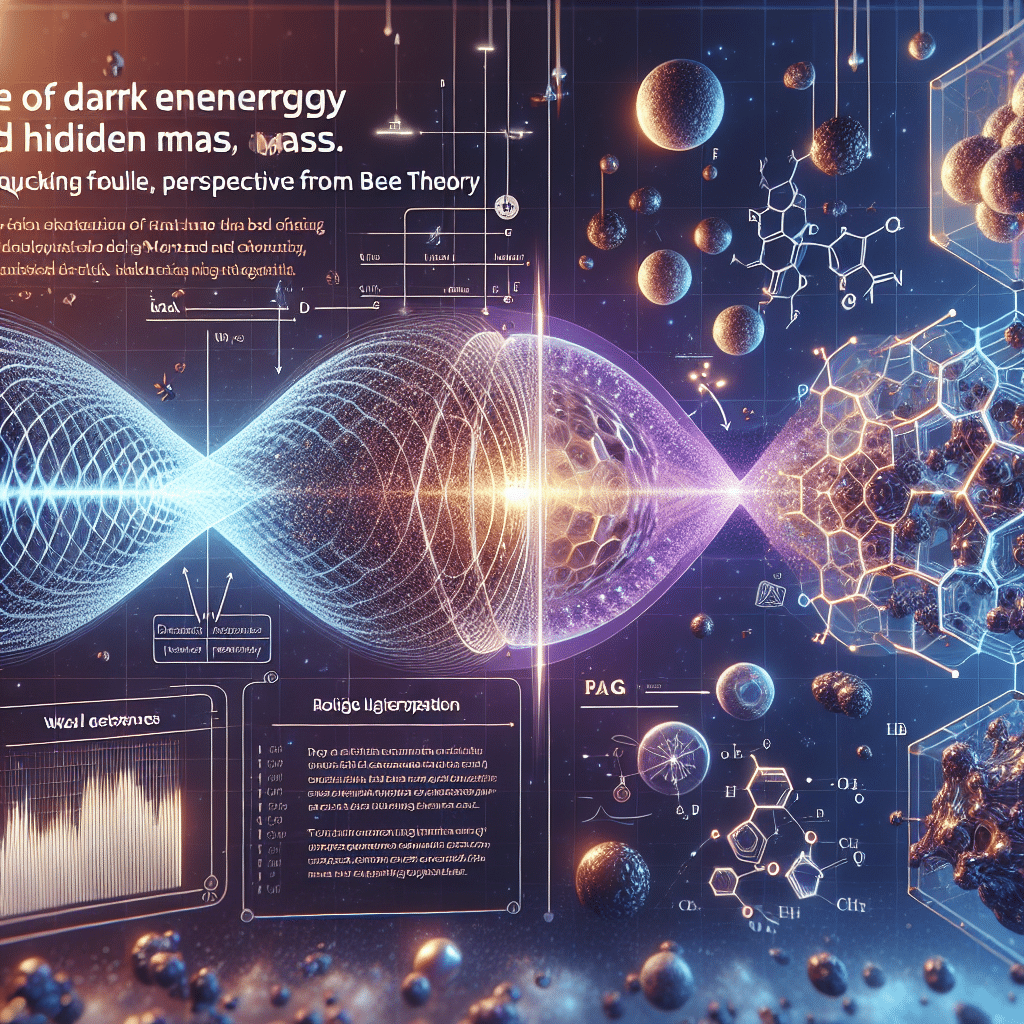
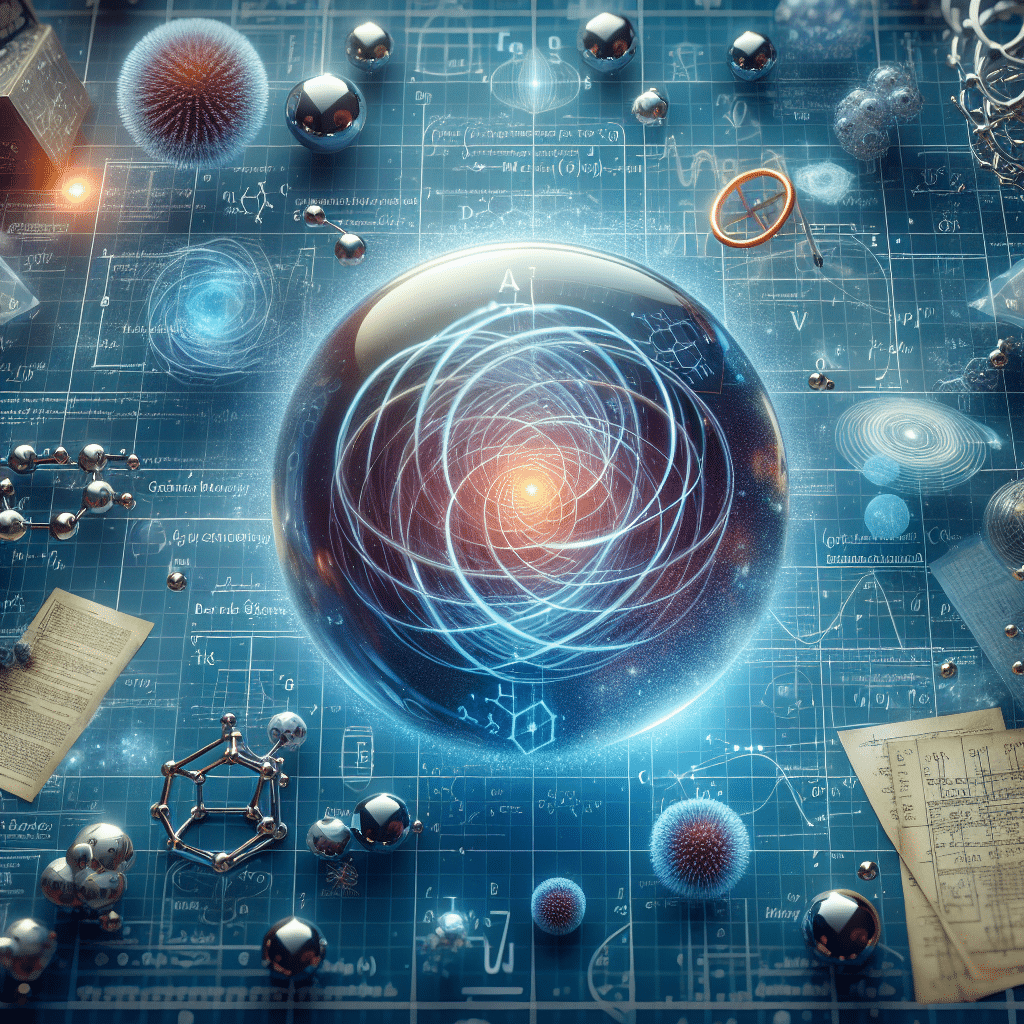
4. Proposed Resolutions and Next Steps
4.1 Refining the Wave-Based Framework
- Derivation of Effective Field Equations
A top priority is a set of effective wave equations that reduce to Einstein’s field equations under low-amplitude or long-wavelength approximations, ensuring Bee Theory aligns with GR in the weak-field limit. Simultaneously, the theory must accommodate phenomena (dark matter, dark energy) without requiring exotic parameters. - Gauge Symmetry and Covariance
Demonstrating covariance under coordinate transformations or an equivalent principle will boost Bee Theory’s credibility. Such a formulation would help unify local inertial frames with wave-based gravitational modes. - Incorporation of Quantum Operators
If Bee Theory is to unify with quantum frameworks, the wave description may need operator formalism analogous to quantum electrodynamics (QED). Introducing “gravitational wave operators” could help describe how these modes interact with standard model particles in a quantized regime.
4.2 Observational and Experimental Validation
- Targeted Astrophysical Surveys
Designing surveys that track specific regions of constructive interference—where wave-based mass effects should be maximal—could provide direct evidence. For example, searching for periodic modulations in rotation curves or lensing distortions would be a critical test. - Next-Generation Gravitational Wave Detectors
Extending detector sensitivity to lower frequencies might reveal persistent wave signals from cosmic-scale oscillations. If Bee Theory is correct, gravitational wave observatories could pick up distinct interference patterns absent in standard GR predictions. - Synergy with Dark Matter Experiments
Direct-detection experiments for WIMPs or axions have yet to yield conclusive results. Bee Theory proponents can leverage these null findings to argue in favor of wave-based gravity. Conversely, if future experiments confirm the existence of dark matter particles, Bee Theory must adapt accordingly, possibly reconciling wave phenomena with particle-based mass contributions.
4.3 Collaborative Approaches
- Interdisciplinary Collaborations
Bee Theory intersects gravitational physics, high-energy physics, computational modeling, and observational astronomy. Fostering collaborative research centers, working groups, and academic programs could accelerate the refinement and testing of Bee Theory. - Open Data Platforms
Sharing high-resolution rotation curves, lensing maps, and gravitational wave data can facilitate independent analyses. Transparency ensures Bee Theory predictions undergo rigorous external validation.
5. Long-Term Vision
5.1 Toward a Unified Physical Framework
Proponents envision Bee Theory as a step toward unified physics—one that might merge classical gravitation, quantum fields, and cosmological observations under a single wave-based principle. This broad ambition echoes the ultimate goal of theoretical physics: a “Theory of Everything.”
5.2 Potential Cosmological Implications
If Bee Theory proves robust, it could reshape our understanding of cosmic evolution—from the early inflationary epoch to the late-time acceleration. It may even provide new insights into phenomena like cosmic voids, large-scale structure filaments, and the distribution of baryonic matter.
5.3 Reconciling with Other Frontiers
- String Theory and Holography
String Theory posits that space-time arises from vibrations of fundamental strings. Bee Theory’s wave emphasis could dovetail with string-based interpretations, but it must be anchored in consistent mathematical underpinnings. - Quantum Entanglement and Gravity
Emergent gravity concepts often tie gravitational dynamics to quantum entanglement patterns. Bee Theory might align with these ideas, suggesting that cosmic wave fields and quantum information are deeply interlinked. - Experimental Metaphysics
In the far future, technology enabling high-precision wave measurements may also illuminate deeper philosophical questions—like the role of information in the fabric of reality or the possibility of multi-dimensional wave phenomena that transcend standard 4D space-time models.
6. Conclusion
Bee Theory presents a bold re-envisioning of gravity—shifting from a purely geometric field to a wave-based phenomenon that could unify dark matter and dark energy within a single framework. Despite its promise, Bee Theory faces substantial theoretical and observational critiques:
- Mathematical Rigor: It must match the precision of General Relativity and quantum field theories.
- Experimental Compatibility: Its predictions should not conflict with well-tested regimes (Solar System, binary pulsars, gravitational wave signals).
- Future Data Needs: Confirmation hinges on advanced surveys, next-generation instruments, and global scientific collaborations.
Addressing these challenges requires a rigorous technical roadmap—complete with robust field equations, gauge-invariant formulations, and a synergy between theoretical development and observational campaigns. If Bee Theory overcomes these hurdles, it could transform our comprehension of the cosmos, offering a cohesive explanation for phenomena that have puzzled scientists for decades. If it falls short, the pursuit itself will deepen our collective understanding of gravity and the profound mysteries that lie at the heart of modern physics. The future of Bee Theory thus remains an exciting realm of scholarly debate, scientific exploration, and innovative thinking—the very ingredients that drive theoretical physics forward.