Masses of Main Quantum Particles in Ascending Order
Particle | Type of Particle | Mass (u) | Mass (MeV/c²) |
---|---|---|---|
Photon | Boson de jauge | 0 (sans masse) | 0 |
Neutrino électronique | Lepton | < 0.0000022 u | < 2.2 eV/c² |
Quark up | Quark | ~0.0000022 u (variable) | 2.2 MeV/c² (variable) |
Électron | Lepton | 5.485 x 10^-4 u | 0.511 MeV/c² |
Neutrino muonique | Lepton | < 0.00017 u | < 170 keV/c² |
Quark down | Quark | ~0.0000047 u (variable) | 4.7 MeV/c² (variable) |
Neutrino tauique | Lepton | < 0.0182 u | < 18.2 MeV/c² |
Quark strange | Quark | ~0.000096 u (variable) | 96 MeV/c² (variable) |
Quark charm | Quark | ~1.275 u (variable) | 1275 MeV/c² (variable) |
Quark bottom | Quark | ~4.18 u (variable) | 4180 MeV/c² (variable) |
Proton | Baryon | 1.007276 u | 938.272 MeV/c² |
Neutron | Baryon | 1.008665 u | 939.565 MeV/c² |
Atome d’hydrogène | Atome | 1.007825 u | ~938.783 MeV/c² |
W boson | Boson de jauge | ~80.379 u | 80379 MeV/c² |
Z boson | Boson de jauge | ~91.1876 u | 91187.6 MeV/c² |
Quark top | Quark | ~173.1 u (variable) | 173100 MeV/c² (variable) |
Higgs boson | Boson scalaire | ~125.10 u | 125100 MeV/c² |
Quantum Particles and the Structure of Matter: An In-Depth Exploration
1. The Role of Gauge Bosons in Mediating Fundamental Forces
Electromagnetic Interaction and the Photon
In the realm of particle physics, gauge bosons play a crucial role in mediating the fundamental forces. The photon, a massless particle and the quantum of light, is the carrier of the electromagnetic force. This interaction governs the behavior of charged particles and underlies essential phenomena like light propagation, magnetic fields, and electrical forces. The photon’s massless nature enables it to travel at light speed, making it unique among gauge bosons and pivotal in facilitating the electromagnetic force.
Weak Nuclear Force and the W/Z Bosons
Unlike the photon, W and Z bosons have mass and are responsible for mediating the weak nuclear force. This force operates over very short distances and is fundamental in radioactive decay processes, where particles like neutrons convert to protons through beta decay. The massive nature of W and Z bosons restricts the weak force’s range, yet it is essential in processes that fuel stars and enable heavy element synthesis.
Symmetry and Force Mediation
The existence of these force carriers is rooted in gauge symmetry, a foundational concept of the Standard Model. Gauge symmetries, specific mathematical principles describing particle behavior, necessitate the existence of bosons like photons, W, and Z particles to mediate interactions. These symmetries enforce conservation laws and dictate interaction strengths, framing our understanding of the forces that govern the quantum world.
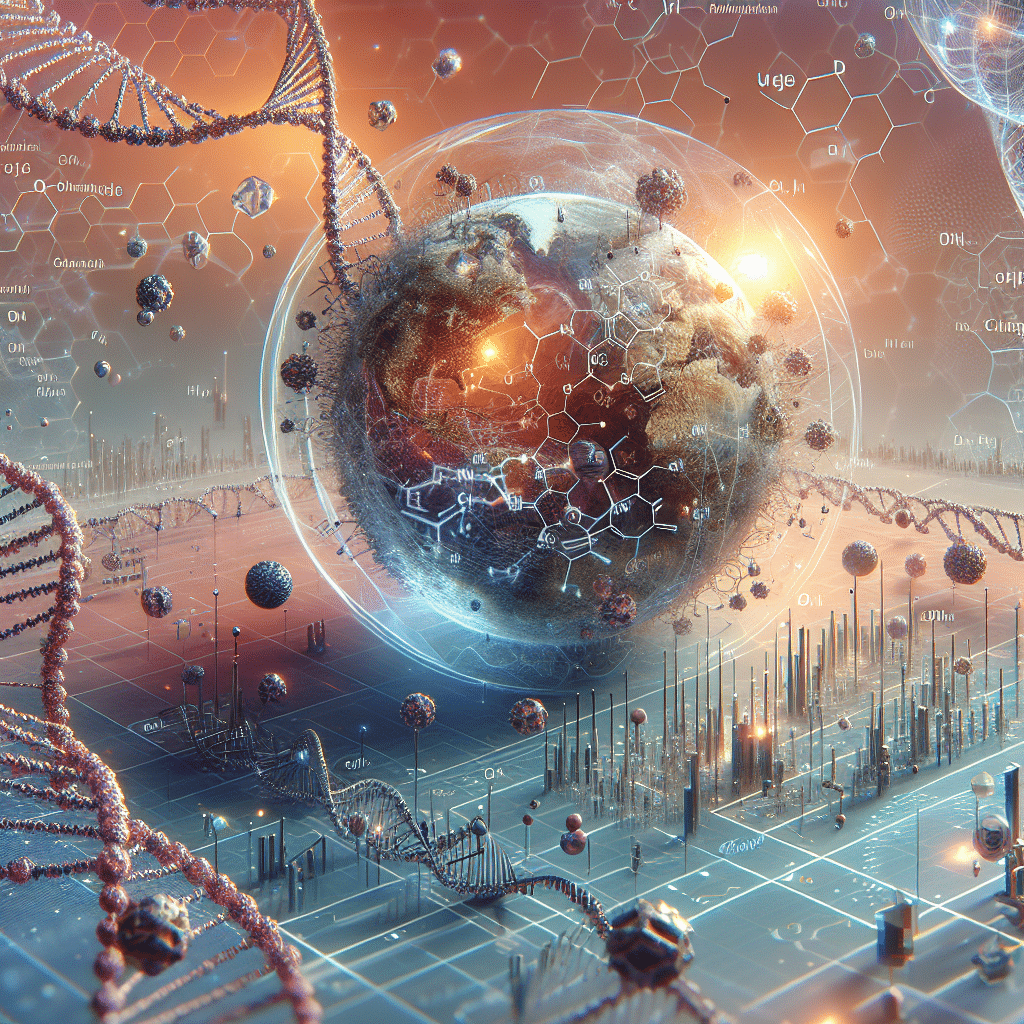
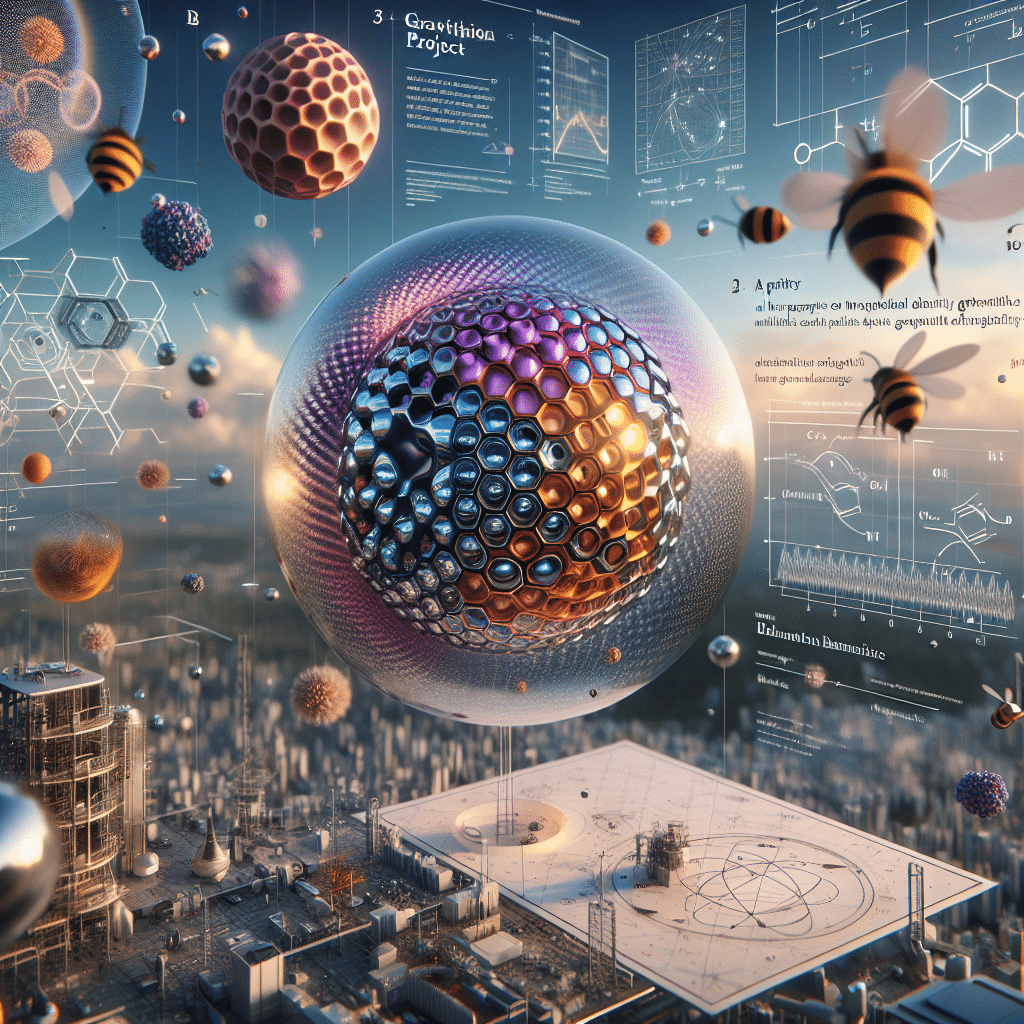
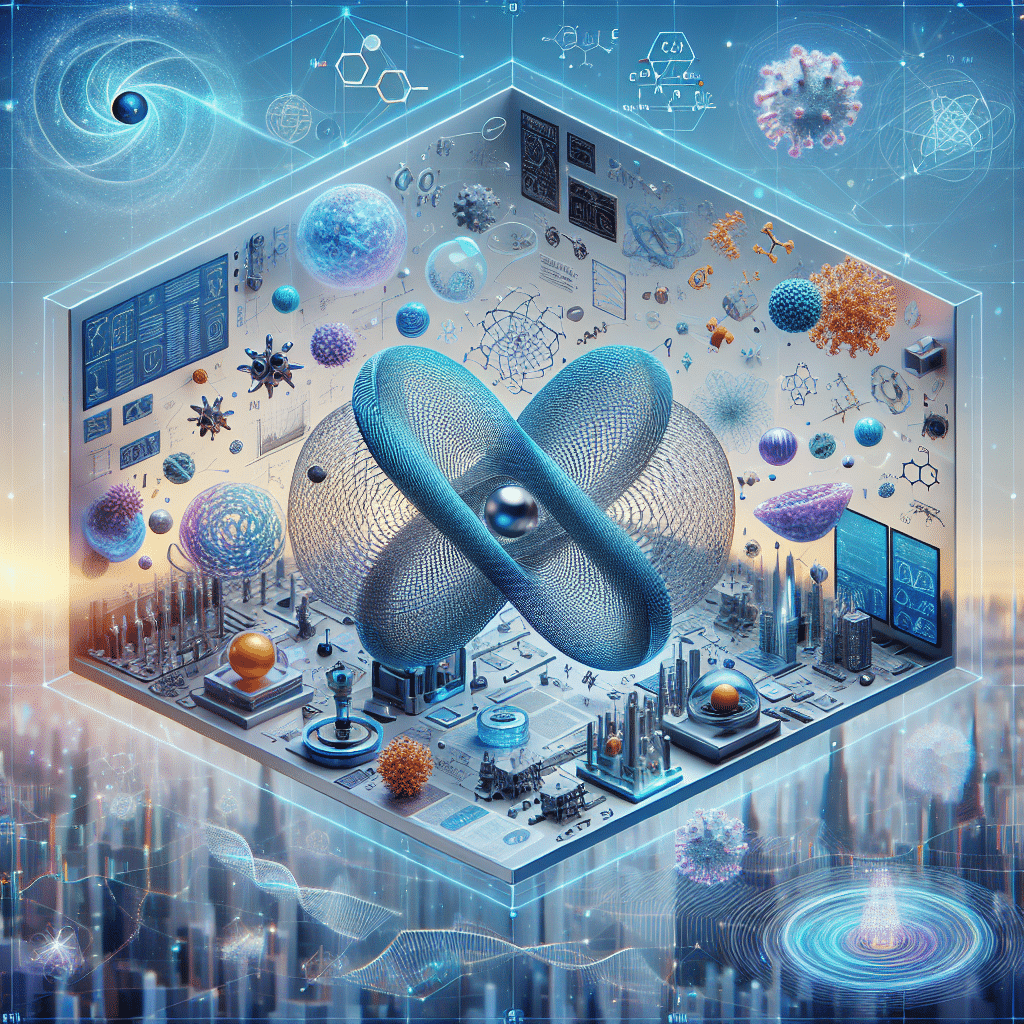
2. Mass Hierarchy and Structure of Matter
The Higgs Mechanism and Mass Acquisition
A cornerstone of modern physics, the Higgs mechanism, explains how particles acquire mass. Through interactions with the Higgs field, particles gain inertia, with the Higgs boson as the field’s quantum representation. This mechanism clarifies why W and Z bosons are massive, while the photon is not, providing essential insights into mass differences among particles and validating predictions within the Standard Model.
Comparing Lepton and Quark Masses
Quarks and leptons exhibit an extensive range of masses, from the near-zero mass of neutrinos to the hefty top quark. Leptons like electrons and neutrinos vary significantly in mass and stability, affecting their roles in atomic structure and particle interactions. The vast mass disparity among quarks, especially the top quark’s large mass, indicates that these particles undergo varying levels of interaction with the Higgs field, directly influencing their stability and presence in high-energy environments.
Mass and Stability of Composite Particles
The combined mass of quarks, governed by strong interactions, underpins the stability of baryons like protons and neutrons. This stability is critical, as it allows baryons to form atomic nuclei, which in turn make up the elements that constitute matter. Protons and neutrons are held together by the strong nuclear force, mediated by gluons, allowing for the formation of stable nuclei and, ultimately, atoms. This hierarchical mass and stability structure shapes the very fabric of all visible matter in the universe.
3. Quarks, Leptons, and the Building Blocks of Matter
Leptons and Weak Interactions
Leptons, including electrons and neutrinos, are fundamental in weak interactions. Neutrinos, particularly, interact only through the weak nuclear force and gravity, making them elusive and challenging to detect. Their interactions drive crucial processes like neutrino oscillation, where neutrinos switch between different “flavors” (electron, muon, and tau neutrinos). These weak-force interactions are vital to understanding particle decay and conservation laws within nuclear and astrophysical processes.
Quark Confinement and Hadron Formation
Quarks are subject to a phenomenon known as confinement, which prevents them from existing in isolation. Instead, they bind together through the strong nuclear force to form hadrons, including baryons (like protons and neutrons) and mesons. Quark confinement and hadron formation are integral to matter composition, with gluons mediating the strong force that binds quarks in stable configurations. This binding is so powerful that quarks remain locked within composite particles under normal conditions, forming the stable atomic nuclei essential for matter.
Generation Structure of Particles
Quarks and leptons are organized into three generations, each with increasing mass and stability variance. While the first generation — up, down quarks, and the electron — comprises all stable matter in the observable universe, the second and third generations feature heavier, less stable particles. These heavier particles typically appear only in high-energy processes and decay rapidly into lighter particles, but they are essential to understanding matter-antimatter asymmetry and particle interactions in extreme environments like particle accelerators and early-universe conditions.